

The surface tension and thus the volume must be kept within a limited range, robots beneath it being too rigid and brittle to control, and ones above it too large to maintain integrity but within this range the fluid exhibited remarkable shape flexibility, including changing aspect ratios and expanding its surface area by a factor of approximately 36 ( Figure 3b, Video S5 in the Online Supplement). The morphological diversity of the fluid robot is limited mainly by surface tension, which depends on volume and composition and could be readily programmed by proper fluid assembly. This enables the tail fluid to physically climb on top of the head, creating the hump, which was consequently prominent when acceleration was high ( Appendix 2).Ī fluid can change shape in ways that are impossible for living organisms, but may be highly desired in various settings. Our video analysis showed that the tail (the rear part relative to the motion vector) remains consistently fluid throughout the experiment and continuously moves towards the head, which compresses and thickens as a result. Analysis of robot locomotion using side-mounted cameras revealed a distinct behavior observed in all experiments, involving the robot's leading end ( head) assuming a hump shape, sometimes up to 10-fold higher than the robot's tail ( Figure 3a). Although the drag coefficient is not constant, our measurements were in good agreement with an approximation of the drag equation based on constant C D ( Table 2 in Appendix 2). This was confirmed by our measurements, in which the reduction in velocity of the loaded robot is visible ( Figure 2e, Video S4 in the Online Supplement).

Our calculations yielded a drag force of 0.07 N, which (assuming an object-to-surface kinetic friction of ≈0.4, and for a robot of the size used here and of the same colloidal dispersion) is sufficient to carry objects of ≈176 g-almost 6-fold heavier than the robot itself. Our initial studies showed clear reduction in the velocity of the robot upon contact with the target weight (e.g., from 1 cm/s to 0.32 cm/s), indicating that a viscous drag force is created between the robot and the weight. We therefore investigated the ability of the fluid robot to carry loads that are significantly heavier than itself-in this case, metal weights. Most robots are designed with the ability to do some form of mechanical work. In the present study we designed a non-Newtonian fluid robot and studied its responses in various programming contexts. Despite that, as in other fields, incompleteness of theoretic understanding does not prevent successful implementation hence, it is possible to assemble specific components to yield a non-Newtonian fluid with the desired properties in a manner analogous to programming a robot for a specific purpose. The physics underlying properties of non-Newtonian fluids, such as shear thinning or thickening, is still only partly understood. However, in contrast, non-Newtonian fluids exhibit diverse, peculiar behaviors deriving from the nonlinear relation between fluid deformation rate and shear stress, which is particular to each system. The mechanical properties and behavior of Newtonian (or approximately Newtonian) fluids, such as water under standard conditions, are largely invariant under applied forces and most other environmental cues. Most importantly, reliable and reproducible control of unbound fluids at high Reynolds numbers is extremely challenging. Some properties required of a robot, such as motion and the ability to perform mechanical work, are natural to fluids others, such as automatic actuation in response to cues, are less trivial.
#Non newtonian fluid skin
Free of constraints such as skin or obligatory structural integrity, fluid robots represent a radically different design that could adapt more easily to unfamiliar, hostile, or chaotic environments and carry out tasks that neither living organisms nor conventional machines are capable of.
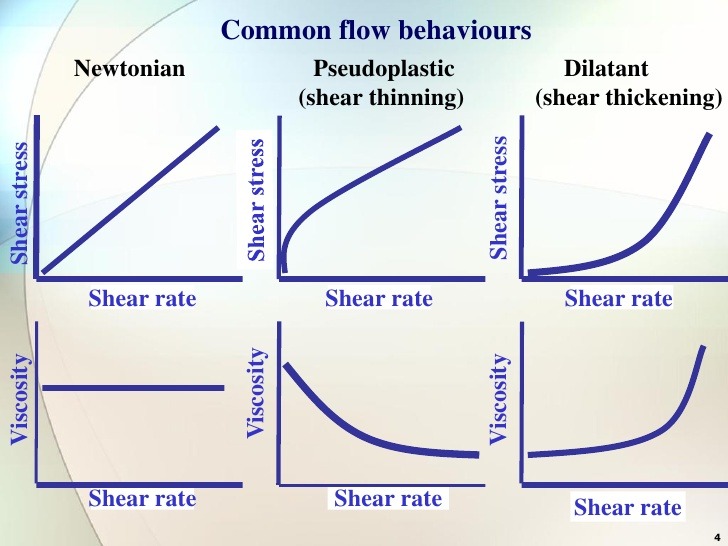
We also utilized interactions between multiple robots carrying chemical loads to drive a bulk chemical synthesis reaction. We demonstrate various robotic primitives such as locomotion and transport of metallic loads-up to 6-fold heavier than the robot itself-between points on a surface, splitting and merging, shapeshifting, percolation through gratings, and counting to 3. We report a robot made entirely of non-Newtonian fluid, driven by shear strains created by spatial patterns of audio waves. In this work we explored the concept of robots that are based on more fundamental physical phenomena, such as fluid dynamics, and their potential capabilities. New types of robots inspired by biological principles of assembly, locomotion, and behavior have been recently described.
